Ricardo Puebla
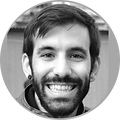
During my research career I have worked on several topics that can be gathered within the broad field of quantum science and technologies. Below you can find a summary of some areas in which I am more interested!
Quantum Control

The external control of a quantum system while preserving its delicate coherent properties is one of the main challenges in the development of quantum-based technologies. The control of a quantum system has therefore applications in a wide variety of problems, ranging from quantum adiabatic computation to quantum gate realization, or the exploration of more fundamental questions in quantum many-body systems. In the context of quantum control, shortcuts to adiabaticity stand out as they have enabled to retrieve the adiabatic transformation of a quantum state but in a finite time at the expense of introducing additional control interactions in the system or leading to a complicated functional dependence of the control with respect to time.
This is one of the most promising areas of quantum-based technologies. Quantum devices may act as sensors with an unprecedented spatial resolution, while at the same time may profit from an enhanced sensitivity to external disturbances. Nitrogen-vacancy centers in diamond are the leading candidates to achieve a quantum advantage in sensing thanks to its good coherent properties, readout and control even at room temperature. Such NV centers act as a quantum register which can be coupled with surroundings unpaired electrons and nuclei: Through sophisticated pulse sequences, one may couple a NV with a targeted element while preserving coherence. Moreover, Bayesian parameter estimation helps in bridging the complex functional dependence between inferred physical parameters and measured data.
Quantum Sensing and Metrology
Quantum Thermodynamics
How do the laws of thermodynamics modify as the size and number of constituents are reduced? Even more, what happens to the second law when quantum fluctuations become significant? These are just an example of the fundamental questions that have attracted a considerable attention in the last decade, and that we can frame within the new field of quantum thermodynamics. In a similar manner as how the are of thermodynamics emerged, these above-mentioned fundamental questions go hand in hand with more applied subjects, such as the inspection of efficiency and performance of quantum thermal engines and quantum batteries, and whether quantum coherence poses any advantage.

Quantum Criticality
Gas, solid and liquid are the well-known phases of matter that we observe in our daily life. Yet, there are other more exotic phase of matter which follow from a precise quantum description of interactions among quantum registers. In the same manner as their classical counterparts, these quantum phase of matter are separated by phase transitions, which take place at the ground state of a quantum system.
The paradigmatic example of a many-body displaying a quantum phase transition is the Ising model with transverse field, where several theoretical developments have been tested. These quantum many-body system become more critical as the number of constituents grow. To the contrary, there are systems where criticality appears as the system parameters are varied, while keeping the same number of constituents. This is the case for the celebrated quantum Rabi model.
Quantum Networks
A quantum network refers to an architecture where several spatially-separated nodes are linked through quantum communication channels. Each of these nodes may contain one or more quantum information units, which can be controlled locally. Superconducting qubits (nodes) and waveguides (quantum communication channels) have already been shown to be a promising platform to realize quantum networks. In this platform, travelling single photons appear as the mediator of quantum information between nodes allowing for a fast communication while suffering minimal losses. Distributed quantum computation or quantum communication protocols could then be carried out within these quantum networks, thus paving the way for a scalable architecture.
In this context, it is essential to design protocols to generate universal quantum gates with high fidelities and that are robust against potential imperfections, as well as to unveil fundamental mechanisms which may limit perfect fidelities. For more information on my activity on this area, see Phys. Rev. Applied 17, 054038 (2022) and arXiv:2212.04899.

Quantum State Engineering
Coherence is a delicate property of quantum systems. Yet, many applications harnessing quantum effects require the preparation of highly coherent or entangled states. Hence, the initial step consists in the preparation of a particular quantum state. In general, quantum state engineering refers to realizing a targeted state by means of interactions or controls at hand. This is an active area of research, which has a natural connection with quantum control. This may be relevant to generate non-Gaussian states (such as cubic-phase or GKP states), or maximally entangled states (e.g. GHZ).